Ca2+-powered stalk contraction of Vorticella
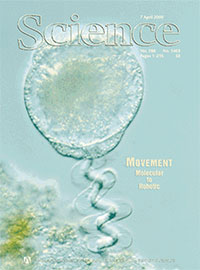
Vorticella is a unicellular animal living in water with its stalk root fixed on a solid surface. Its inner stalk contractile organelle, the spasmoneme enables Vorticella to contract in less than 10 msec. Because the maximum speed is in the order of 1 cm/sec and the spasmoneme generates force up to 300 nN, Vorticella is a model for biomimetic actuators being one of the fastest and powerful cells.
Another unique feature of Vorticella is that its stalk contraction is powered by calcium ions, not ATP (adenosine triphosphate, a universal biochemical fuel in biological systems). Although the spasmoneme used to be regarded as a primitive type of muscle, it is now believed that the spasmoneme has a different contraction mechanism from muscle because the spasmoneme converts chemical energy from calcium to mechanical work. Therefore, Vorticella serves as a model system for Ca2+-powered cell motility.
Our research on Vorticella is focused on investigating the contraction mechanism of Vorticella and developing biomimetic engineering systems inspired by Vorticella.
(Image: Contracting Vorticella: Cover page of Science)
Microfluidic mixer mimicking Vorticella
One of the reasons suggested for stalk contraction of Vorticella is that Vorticella augments mixing (for more food resource) around its habitat by stalk contraction. After ultrafast stalk contraction, the stalk of Vorticella slowly relaxes to its extended state, which takes a few seconds. Therefore, the Reynolds numbers of contracting and relaxing Vorticella is greater and much smaller than the unity, respectively. This means that flow induced by stalk relaxation is viscous force dominated (creeping flow) whereas both inertia and viscous forces are important in flow induced by stalk contraction. Vorticella seems to take advantage of this difference in flow characteristic seems to enhance mixing around it. Inspired by the mixing mechanism, we aim to develop a microfluidic mixer mimicking the stalk contraction-relaxation cycle of Vorticella.
Biomimetic Ca2+-powered polymeric actuator
Certain microorganisms have Ca2+-powered contractile organelles for their motility, and well-known examples include the spasmoneme of Vorticella and the infraciliary lattice of Paramecium. Similar organalles are also found in eukaryotic cells, and the microtubule organizing center of human cells is an example. The common feature of these organelles is that they consists of filaments of nanometer diameter, and these filaments contain Ca2+-binding proteins and their binding partners. It is believed that these filaments coil or fold upon Ca2+-binding converting chemical energy to mechanical motion. Inspired by this contraction mechanism model, we plan to develop a biomimetic polymeric actuator converting chemical energy from calcium to mechanical energy by reconstructing Ca2+-responding nano-filaments in vitro using Ca2+-binding proteins and their respective binding partner proteins.
Scaling law and pinch-off in soap bubble generation
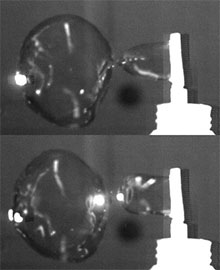
Although very common, soap bubbles are still fascinating people regardless of their age. It is not only a very simple scientific experiment but also a very fun activity to blow soap bubbles with a frame to make soap film from a soap solution made of water and surfactant (for a quick example, dish washing liquid). However, it is little understood how three dimensional soap bubbles generate from two dimensional soap film expanded by air flow.
Soap bubbles are different from liquid drops in air or gas bubbles in liquid because they are like a very thin liquid structure seperating inner-bubble gas from outer-bubble gas. Therefore, soap bubble generation poses challeging questions in surface-tension-dominated interfacial fluid dynamics. Questions are, for instance, what factors govern the size of soap bubbles, what induces fluid dynamic instability of soap film, and how soap film pinch-off occurs.
To tackle these questions, we plan to develop a jet flow apparatus to mimic human soap bubble blowing and aim to discover the scailing law among factors governing soap bubble size. In addition, we will control and visualize air flow expanding soap film pocket and presumably inducing instability of the pocket to investigate how interaction between soap film and air flow plays a role in soap bubble generation.
(Image: Soap bubble pinch-off: Courtesy of John Davidson)
Wall effect at the Reynolds number ≥ 1
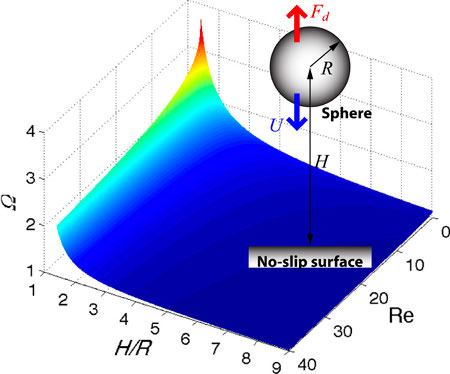
When a particle moves toward a no-slip surface, the particle experiences drag force higher than drag that it experiences in an infinite fluid. This increase in drag due to the surface is called the wall effect, and the wall effect is known analytically for the spherical particle moving very slowly in a highly viscous liquid, i.e., for the Reynolds number much smaller than the unity (Re << 1).
In contrast, the wall effect for Re ≥ 1 is rarely studied although particles happen to move toward the wall with significant speeds in the cases of heavy sedimenting particles and contracting Vorticella. To predict motion of such high speed particles, it is crucial to understand the wall effect for Re ≥ 1. Therefore, we aim to experimentally measure drag force on the sphere and cylinder moving toward a wall at Re ≥ 1, to investigate induced flow field, and to suggest empirical correlations for the wall effect.
(Image: Sphere moving toward the wall, and wall effect factor as a function of Re)
Rupture dynamics of focal adhesion
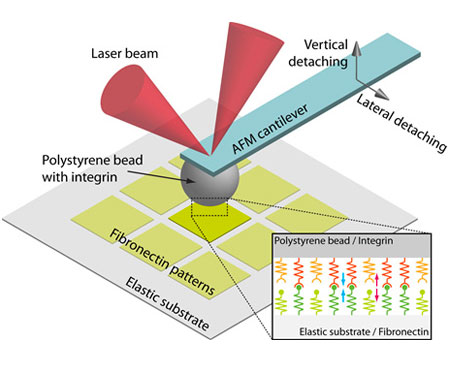
Cells interact with their extracellular matrix (ECM) through focal adhesions, anchor-like protein structures on cell membrane. Via focal adhesions, for instance, cells not only exert traction forces to the ECM, but also sense changes in mechanical properties of the ECM. For better understanding of cell-ECM interaction, therefore, it is required to investigate how focal adhesions respond to mechanical stimuli applied to them.
For this purpose, we developed an in vitro focal adhesion system mimicking focal adhesions. The system consistns of a colloidal AFM probe coated with intergrin (focal adhesion receptor) and an elastic substrate coated with fibronectin (ECM ligand). By controlling moving speed and direction of the probe and the stiffness of the substrate, we will investigate how the focal adhesion ruptures responding to changes in its mechanical environment.
(Image: Schematic of the biomimetic focal adhesion model system)
Further research interests and plans
- Aerodynamics of wind turbine
- Mechanobiology of stem cell differentiation
- Motility of cancer cells